In life, everyone must play with the cards they are dealt and hope for a good hand. Just like the cards that a player holds, one’s inherited genes are powerful indicators of what is in store. This might seem to imply that one’s fate is predetermined, but this is not necessarily the case — after all, games are transient by nature. Genes are subject to change, just like games; they change subtly over time, and eventually, the final product does not remotely resemble the predecessor. Before developing a life-changing treatment, researchers have to ask which genes lead to a disease. The quicker scientists uncover the mystery of genetics, the closer everyone is to understanding how to best play their hand.
At UC San Diego, student researchers attempt to uncover this very mystery in the context of the human heart and the development of cardiovascular diseases. There are a myriad of complications in the heart that can lead to cardiovascular diseases, and the cause of these complications can often be traced back to genetics. As little as one alteration in the genetic “deck” can result in disease. Thus, by studying genetics, researchers are working towards understanding not just the intricate set of rules that govern genetics, but also how to change these rules.
Dealing Cards
Third-year student Anh Nguyen, a volunteer researcher in Dr. Xi Fang’s lab, studies the effects of a mutation in a gene called TAFAZZIN (TAZ), which is expressed in the walls of the heart. A mutation in TAZ is responsible for Barth syndrome, which is characterized by a weakened heart. This mutation leads to defects in the production of cardiolipin, a crucial component of the mitochondria in the cell. Dysregulation of these cardiolipins due to mutations in TAZ results in cardiomyopathy, a disease of the heart muscle. Cardiomyopathic hearts find it more difficult to pump blood to the rest of the body, ultimately leading to more serious complications such as heart failure.
In a healthy heart, the ventricles and the septum, which separate the chambers of the heart, are strong
and thick in order to pump blood to all the extremities. Contrastingly, cardiomyopathic hearts have thinner ventricular and atrial walls, leading to significant weakness compared to a normal heart. Throughout the course of the Fang lab’s research, Nguyen observed that the abnormal production of cardiolipin eventually leads to decreased efficiency at which the heart can pump blood. Since pumping blood is an energy-taxing process, this function cannot be performed without sufficient energy from the mitochondria. While mature cardiolipins regulate the shape of a healthy mitochondria, hearts that lack sufficient cardiolipins because of a mutation in the TAZ gene may possess mitochondria with an onion-like shape. Currently, Nguyen is evaluating whether these unusual, onion-like mitochondria contribute to weaker cardiac functions.
In order to study the effects of the TAZ mutation on cardiovascular disease (CVD), the Fang lab is comparing mice that lack the TAZ gene in heart muscle cells — known as “TAZ knockout mice” — with mice that have the normal TAZ gene. They plan to investigate differences in the production of cardiolipin in heart muscle, heart size, and thickness of the walls at different intervals of time. Nguyen also examined the shape of mitochondria in mice’s hearts using an imaging tool. After two months, knockout mice’s mitochondria were longer and had more variation in size; after four months, they were smaller and longer. The images also captured the onion-shaped mitochondria in hearts that lacked the TAZ gene. Compared to the mitochondria in control mice, mice without the TAZ gene had higher percentages of abnormal mitochondria in size and shape. With upcoming data, Fang’s lab seeks to elucidate and confirm the correlation between the disfigured mitochondria and cardiomyopathic hearts. The current running hypothesis is that a deletion of the TAZ gene in cardiomyocytes of mice has resulted in dilated cardiomyopathy (DCM). DCM is a condition in which the left ventricle walls thin and the ventricle enlarges, preventing the heart from pumping blood efficiently. With this confirmation, the lab opens the possibility of future DCM treatments that save lives by adjusting a gene.
Ordering the Cards
The genes each of us are dealt are influential health factors. While it may seem that each person is entirely genetically unique, a lot of our genetic makeup is shared with people to whom we share no relation. These genes are often found in non-coding regions. But what are noncoding regions?
Each individual’s genetic information contains unique codes that tell the body what proteins to make. Every single code is important — either explicitly or implicitly. Coding regions are portions of DNA that are directly transformed into amino acids and proteins. Vivian Phan, a third-year human biology major researching in Dr. Sebastian Preissl’s lab, instead analyzes non-coding regions in cells. These are portions of the genetic code that are not transformed into proteins, making their importance and role more hidden and elusive.
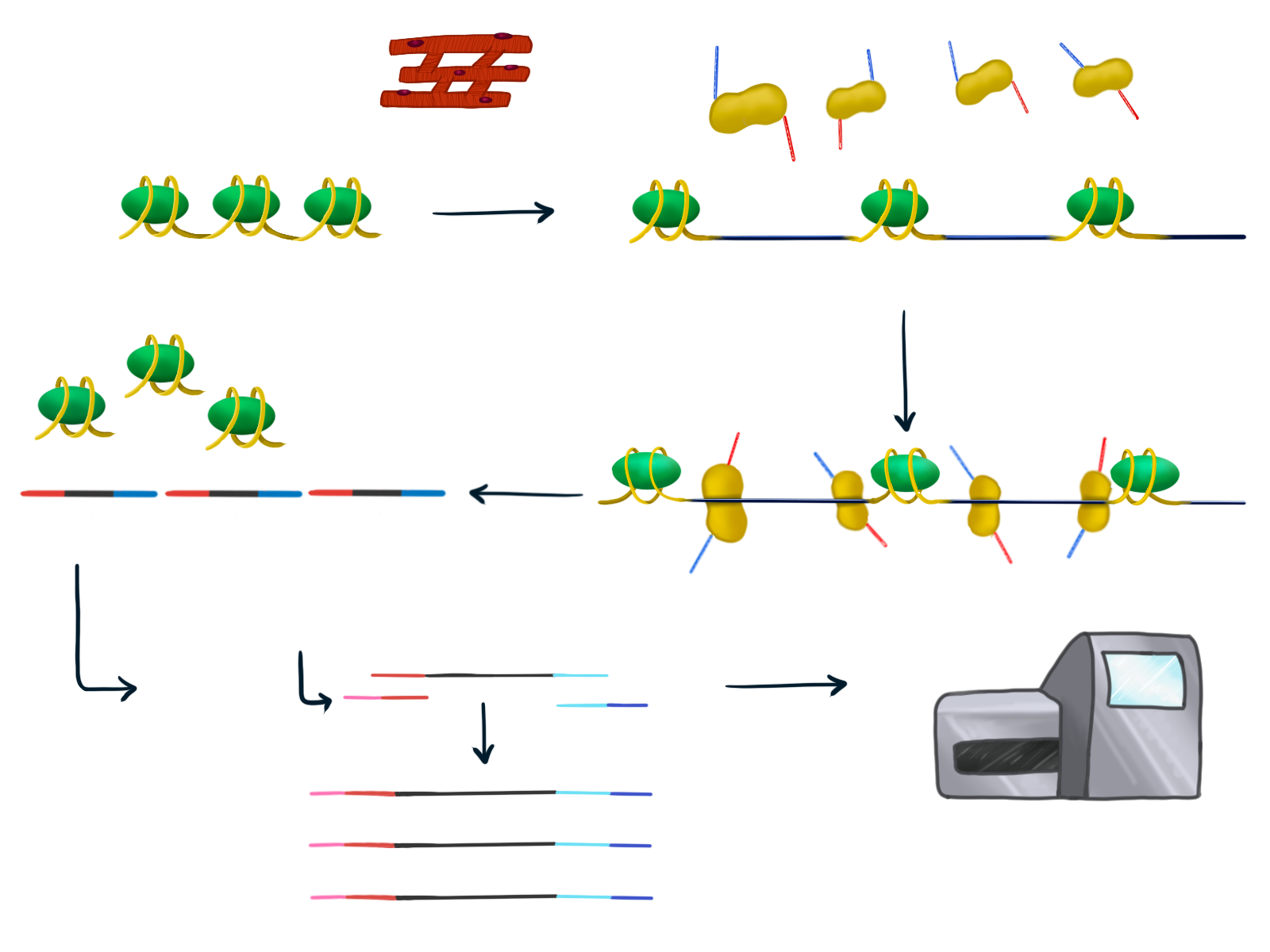
Though non-coding regions may not seem directly important, they regulate how much of a protein is produced and where it is produced, according to fellow Preissl lab member Jake Hocker. Enhancers are a type of non-coding region which “have an active role in regulating the expression of genes nearby on the same DNA sequence,” says Hocker, who argues that detailing enhancer regions can clarify “which proteins bind to those regions to control what happens in the disease.” Enhancers are short strips of DNA that tell proteins to start coding.
By detailing these regions, Phan “hope[s] to use single cell analysis to reveal transcriptional regulators of gene expression programs in heart failure,” particularly changes that lead to ischemic heart disease, a type of CVD where blood flow is impaired due to the narrowing of blood vessels. Phan hopes that eventually, this could lead to viable treatment options.
Uncovering New Cards
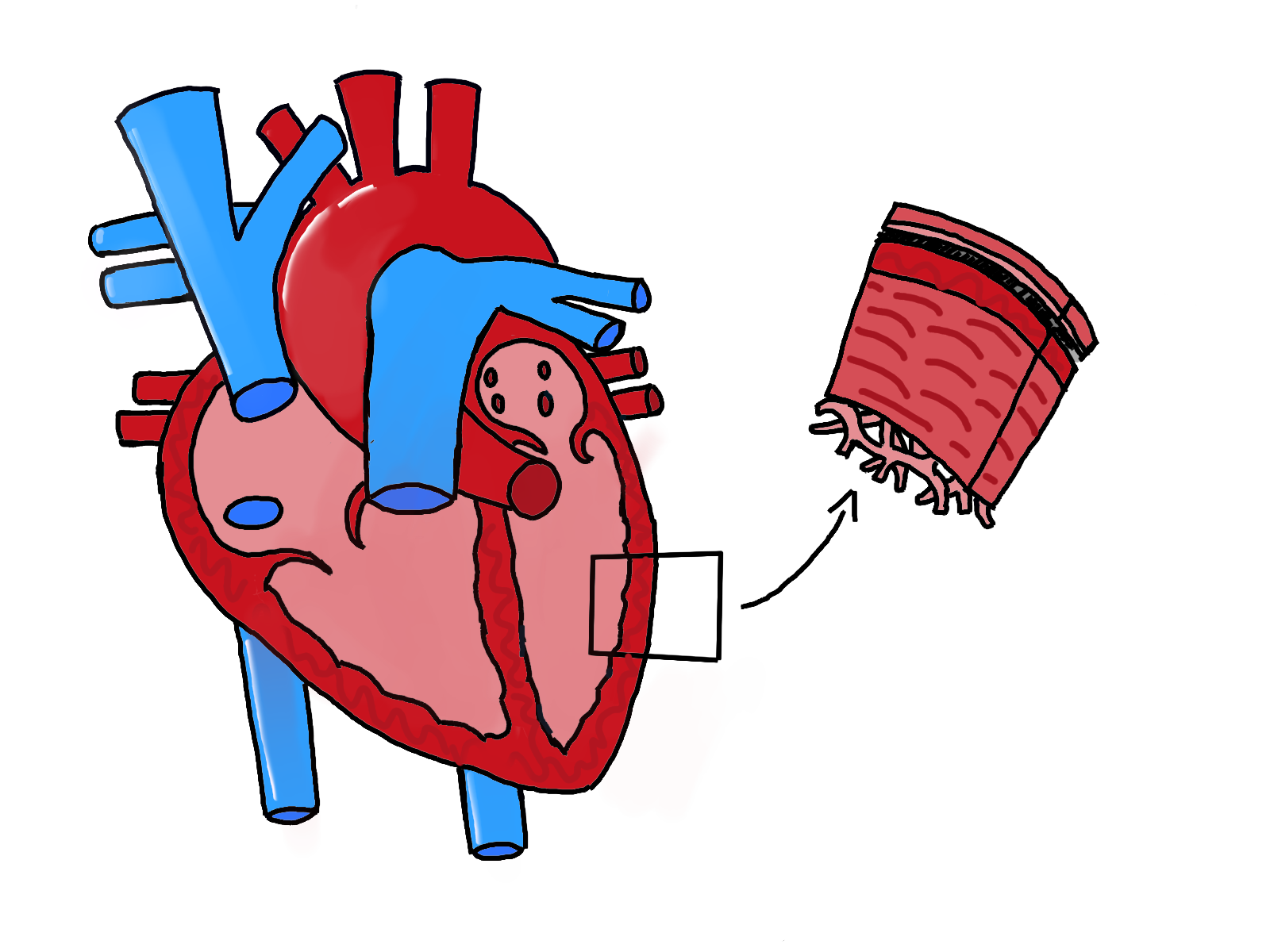
When playing solitaire, cards are strategically placed in a predetermined order, laying out the deck in a way that is easy to the hand pile survey. At the start of the game, this may appear to be a lofty and near-
impossible goal; however, when it seems all hope is lost, there is always hand pile, capable of furthering the game and revealing more secrets lying hidden within the deck. Once the player gets going, the pattern slowly reveals itself. A similar method of analysis on single cells is performed in the Preissl lab to display their genetic deck.
Phan and Hocker’s method of choice for analyzing these open chromatin regions is ATAC sequencing, a method of profiling the chemical DNA alterations that are not reflected in the actual sequence. First, they obtain a very small sample of heart muscle from the left ventricle, chosen specifically because this is where the most amount of changes in gene expression occur. This makes it the most common part of the heart that is affected by heart disease. Many changes occur here because some regions of DNA are closed, or unable to be read, whereas others are open so that they are able to be read and expressed. When regions of DNA are open that means they are able to be translated into proteins and therefore have an effect on the development of genetic disease. ATAC sequencing analyzes only the open chromatin regions of DNA.
With their goal of determining which transcription regulators play a key role in heart failure, Phan and Hocker use advanced DNA sequencing methods to determine which regions are open and which are closed. Next, they harvest pools of nuclei, the part of the cells containing DNA, which are placed in a 96-well plate, with each of the wells containing thousands of nuclei. They add an enzyme to each well that cuts up the open chromatin regions and attaches a unique barcode to each one. Then, they mix the contents of all the wells together and conduct PCR, which will attach yet another barcode unique to each well. Now, each of the open regions have a unique barcode combination. Using these unique combinations, they compare gene expression and open or closed chromatin regions from samples of hearts that experienced heart failure to those that did not.
This method of analysis allows the Preissel lab to determine where each sample came from and which cells they belong to. In the past, labs have taken samples from many different regions of the heart and analyzed them all together, leading to certain cell types being overrepresented. However, by only taking cells from the left ventricle, the Preissel lab will be able to more easily determine where the open chromatin regions are from and what their role is in the development of heart disease.
Research on cardiovascular disease on the single-cell scale has not yet been done. “I like how the field is constantly changing and evolving,” says Phan, stating that though there are a lot of unknowns in this area of research, it is a promising approach to discover more about these diseases.
Winning the Game
Just as card players must find a winning strategy from their own hand, CVD can only be addressed by examining each individual’s genome. The prevalence of CVD research has expanded from industrialized
nations like the United States, United Kingdom, and Australia to middle and low income countries. With each discovery on the genetic foundations of CVD, scientists gradually come closer and closer to developing treatments for heart-related disease and perfecting gene corrections using gene therapy. Certainly, there continue to be technical issues with the application of gene therapy since it is relatively new, but as the Fang and Preissl labs identify areas for future CVD treatments, it seems there are paths to victories in this game of life, so long as we learn to take them.
Written by Rachana Balakrishnan & Leanne Dugan
Rachana is a first year student majoring in Neurobiology. Leanne is a third year student majoring in General Biology.