Old body parts, fluids, and an exploration in alchemy become a reality in Frankenstein, when a grotesque creature is brought to life from inanimate materials. Throughout the story, the monster struggles with his own “biologically-engineered” identity–is he really human?
Biologically speaking, Frankenstein’s monster is of course a stretch. But, if we can get past the alchemy, other parts of his experiment illustrate the possibilities of chemical concoctions on human life. One of the most important ingredients of the experiments undertaken in Victor Frankenstein’s sequestered laboratory is the mystery “solution” in which the monster’s misconstrued body parts are seemingly brought to life.
In today’s world, Frankenstein’s monster may have been held together by hydrogels, 3-dimensional networks formed from synthesized polymers. Hydrogels are as powerful as they are complex: structurally robust due to the cross-linking of ion-rich polymers, but elastic due to their polymers’ hydrophilic (water-loving) nature. Hydrogels are also responsive to stimuli–adjusting to changes in surrounding pH and temperature either to stay the same or change entirely in structure. With the right combination of thermodynamic conditions, reaction-induced molecular interactions, and fabrication methods, a mixture of distinct macromolecules gives way to a miraculous product, almost as promising as Frankenstein–a hydrogel.
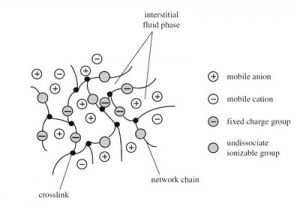
Since their inception in the 1960s, hydrogels have demonstrated great utility in enhancing human life. In a small Czech town located on the outskirts of Zlin, Otto Wichterle had already established himself as a leader in the field of chemistry by developing polyamide and caprolactam plastics, as well as being the first to author a formal textbook on inorganic chemistry. His vision for plastics was much loftier, presumed from his fiery gaze through his clear-framed glasses. Wichterle believed his inorganic compounds could interact with biological structures on a nano-scale, a concept far ahead of his time (this field would later become known as biotechnology). As a man suffering from vision deficits himself, he set out with the goal of developing a substance that could conform to the delicate environment and conditions of the eye. With the help of his wife, Wichterle succeeded in creating the world’s first set of contact lenses by synthesizing a new substance which he called a “hydrogel” on his kitchen table. On Christmas Day of 1961, Wichterle carefully tried on his prototype, seeing for the first time a world more focused than his own vision of hydrogels. For the first time, man and microscopically-complex structures were fused together, an indiscernible combination from the outside.
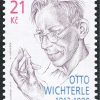
Wichterle’s solution is a powerful illustration of what hydrogels mean for us. The versatility and adaptability of these structures–robust yet elastic, dynamic yet stable–provide a more advantageous solution to many medical problems. Our gut instinct at solving bodily deficiencies, vision in this case, is to create mechanical solutions which exist distinct from our bodies (i.e. glasses). The result is a prototype living a life of its own from our anatomy, which solves the immediate problem, but leaves room for improvement. Instead of innovating solutions to medical problems as accessories existing outside of our bodies, why not innovate for seamless integration with our bodies? In order to “fix” our bodies, we need to create structures that will adapt to their mold, fitting in with the biological characteristics that make us human.
Wichterle’s vision for hydrogels has rapidly become a reality in biological solutions. Hydrogels, for example, can be used to heal damage inflicted during surgery. Adhesions, organ tissue sticking to surrounding tissue, are a common anomaly in post-operative assessment. These adhesions make it difficult to operate on organs again, which poses a problem for patients with systemic heart problems. To address this issue, a group at UC San Diego engineered a hydrogel from an amino acid called L-dopa and a catechol polymer to prevent post-operative adhesion of heart tissue. In porcine (pig) hearts, the gel resulted in fewer adhesions while also not inducing cardiac inflammation.
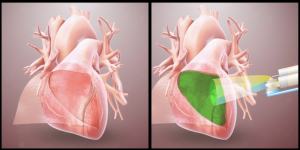
While Wichterle’s creations were molded as temporary fixes, hydrogel innovation evolved to heal the body sustainably. Researchers at Northwestern University developed a gel mimicking the scaffolding structure holding together cells in the spinal cord to repair damage done to local nerves. When injected into paralyzed mice, the gel assembled itself at the injury site and helped regenerate damaged neurons and reduce scar tissue. Furthermore, the gel enabled the growth of nearby blood vessels, providing further support to the damaged site. Amazingly, after four weeks, paralyzed mice treated with the gel could walk again.
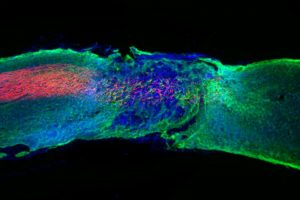
Hydrogels also present a more effective and natural way to deliver drugs within the body. Administering doses of treatments without specific targets decreases efficacy, as cells important to immune response are often killed in the process. To address this issue, hydrogels provide a great drug delivery system, as they are primarily composed of water and structurally crosslinked, which prevents outside proteins that may denature the treatment from permeating through the gel. The chemical structure of the hydrogel may also be changed to address selective targets and to naturally degrade once used.
Carefully toiling away in his laboratory, Wichterle imagined the great potential of hydrogels but likely didn’t comprehend its full capabilities. Hydrogels embrace solutions which combine robust chemical engineering with the biology that makes us human.
References:
- https://www.ncbi.nlm.nih.gov/pmc/articles/PMC4494665/
- https://www.nature.com/articles/ncomms3226
- https://www.gruppotpp.it/en/hydrogel-based-delivery-systems-definition-release-mechanism/
- https://www.degruyter.com/document/doi/10.1515/ci-2015-0241/html
- https://www.thecontactlensmuseum.org/otto-wichterle.html
- https://www.degruyter.com/document/doi/10.1515/ci-2015-0241/html
- https://www.nature.com/articles/s41467-021-24104-w
- https://www.nature.com/articles/s41467-021-24104-w
- https://www.science.org/doi/10.1126/science.abh3602
- https://www.nature.com/articles/natrevmats201671