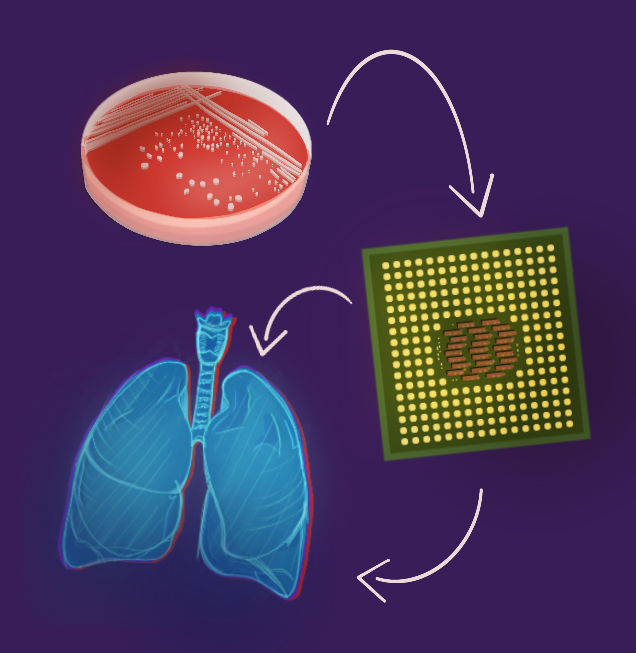
Culturing organ tissue to study the cell is a foundational technique for testing drug toxicity and efficacy. Present in vivo methods, where experiments are performed on animals, are often limited by ethics, cost, low throughput and differences across species. In vitro methods, such as growing homogeneous human cells in a petri dish with growth medium, takes cells out of their endogenous context and limits model accuracy. Although these laboratory practices are widely utilized, both fall short of replicating the diversity of multiple organ systems. The demand for an effective in vitro model could potentially be answered with an emerging technology, the “organ-on-chip”. This tool may allow researchers to emulate and control the complexities of tissue cell environments. The flexibility of these chip-based instruments is a promising addition to the arsenal of tools researchers have at their disposal to find the next drug hit.
The need for an in vitro method more physiologically relevant to the human body to test potential drugs and analyze how human tissue responded to perturbations was clear. This motivated Dr. Donald Ingber’s team at the Wyss Institute at Harvard to build the first lung-on-a-chip in 2010. The team placed a cell culture of lung tissue on a chip with microfluidic channels to create a 3 dimensional model of lung tissue cells. The defining feature of the microchip is that it enables compartmentalization of various fluids and cultures on one dish. Human organs consist of different tissues that do not act in isolation, working together to carry out bodily functions. The microchip connects these tissue networks and could bring research closer to accurate in vitro models.
In the context of cancer, testing potential therapeutics is highly dependent on understanding the tumor environment. In order to do so using a microfluidics chip, a complex and chemically fluctuating network is required to recreate the stages of abnormal cellular mitosis. The tumor microenvironment consists of cancerous cells, immune cells, and connective tissue cells. Additionally, the human body provides a variable source of nutrients and oxygen. The shortcoming of 2D models is that they are monolayered and lack separation between cell culture and extracellular environment. As a result, 2D tissue cutlures lose their original morphology because of interfering external stimuli which affects their method of cellular replication. The rigid and flat surfaces of the 2D chip do not sufficiently provide a stable supply of nutrients. This presents another failure because a source of complex nutrients is required for the cells to mature in a way that properly mimics tumor environment. The key to improving accuracy of these models is to imitate the tumor’s microenvironment in a three dimensional manner. The 3D tumor-chip contains a culture of cancer cells and endothelial cells (membrane lining of heart and blood vessels) in a hydrogel. The device has microfluidics channels which act as energy gradients by using differences in ion concentration, intended to mimic the biological functions of the tumor system. The hydrogel replicates the extracellular environment of the tumor and contains synthetic polymer molecules to provide the culture with nutrients to mature. In contrast to the 2D monolayered cultures, the room for interactions of heterogeneous cells and a diverse supply of nutrients on the microchip may address the challenges of cell maturation and reliability.
Ninety percent of cancer deaths result from a late-stage complication called metastasis, which is the relocation of cancer cells to other organs through the bloodstream. Creating a model of this migration in cell culture can aid the search for drugs to suppress tumor growth. To do so, the hydrogel can be regulated along the chip’s channels to track or block circulating cancer cells. One method involves distinguishing the circulating cancer cells based on shape, size, and antigen marker. The microfluidics chip traps the tumor cells and separates them from blood cells, then filters them through an arrangement of microchannels and applied forces in a non-uniform electric field. By controlling the flow of cells in channels and the porosity of filters, researchers were able to obtain pure circulating cancer cells. This microfluidic filtration is just one of the ways in which the chip has been used to target and examine cancer. While this chip technique is not broadly applied, it demonstrates promise in the field of 3D systems to perform accurately at the organ-level.
One application of the lung chip, which was the first organ mimicked on a chip, is to to study lung cancer. Due to lung cancer’s high mortality rate, researchers were motivated to analyze tissue-tissue interactions and tumor growth in a relevant system. They restricted cancerous cells to specifically controlled areas of the chip and provoked active breath motions to parts of the tissue cells. This involved mechanically stretching the membrane of tissue cells using a vacuum in the microchannels. The basic lung chip led to the observation that cancer cells were attracted to stimulated regions known as the alveolar space. Lung cancer cells appeared to suppress breathing activity, which facilitated cancer growth. Based on prior knowledge of the liver’s role in metabolizing inhaled toxins and drugs, researchers performed a study with a chip containing both the endothelial cells of the lung and liver cells. The goal of this experiment was to observe how the inhaled toxin AFB1, which has shown to be a potential cause of lung cancer, is metabolized by the liver. By compartmentalizing these cells and exposing AFB1 on the chip platform, observations were made on how they interacted with each other. Liver cells appeared to metabolize AFB1 into its detoxified form which decreased the positive feedback of lung cancerous growth. On the basis of these findings from co-cultuiring liver and lung cells, this may aid in the search for drug compounds which enhance liver conversion of AFB1 to possibly mitigate the toxicity which induces lung cancer. The interplay between different organ cell types in this experiment demonstrates the importance of solidifying multi-organs-on-a-chip systems to provide a more comprehensive analysis of drug therapeutics.
Since its first conception in 2010, the organ-on-a-chip has emerged as a promising in vitro method for observing human cells in a tissue context. This tool could more accurately screen potential drugs, and observe factors involved with disease development such as metastasis and tumor cell occupation. As researchers continue to make strides in improving this model, a chip which precisely mimics the network of mechanisms across multiple organs, coined a “multi-organ-on-a-chip”, remains elusive yet necessary. Nevertheless, the technology has become increasingly accessible and diverse. A startup founded by Dr. Ingber and his team, Emulate, advertises six organ-on-a-chip kits such as for the brain, colon intestine, and liver to use in research labs. Its increased application will aid in vaccine testing, studying organ disorders, and creating cancer therapies. The goal is to have a replica of the body with as many biological factors that play a role in disease response, all on a small chip.
References
- Agnieszka Zuchowska, Sandra Skorupska, Multi-organ-on-chip approach in cancer research,Organs-on-a-Chip,Volume 4, 2022,100014, ISSN 2666-1020 https://doi.org/10.1016/j.ooc.2021.100014
- Ribeiro-Samy, S., Oliveira, M.I., Pereira-Veiga, T. et al. Fast and efficient microfluidic cell filter for isolation of circulating tumor cells from unprocessed whole blood of colorectal cancer patients. Sci Rep 9, 8032 (2019). https://doi.org/10.1038/s41598-019-44401-1
- Donald Ingber, Wyss Intitute, Human Organs-on-Chips, https://wyss.harvard.edu
- Liu, X., Fang, J., Huang, S. et al. Tumor-on-a-chip: from bioinspired design to biomedical application. Microsyst Nanoeng 7, 50 (2021). https://doi.org/10.1038/s41378-021-00277-8
- Emulate Website https://emulatebio.com/products-services/
- Kapałczyńska M, Kolenda T, Przybyła W, et al. 2D and 3D cell cultures – a comparison of different types of cancer cell cultures. Archives of Medical Science. 2018;14(4):910-919. doi:10.5114/aoms.2016.63743.
- Bovard, D., Sandoz, A., Luettich, K., Frentzel, S., Iskandar, A., Marescotti, D., Trivedi, K., Guedj, E., Dutertre, Q., Peitsch, M. C., & Hoeng, J. (2018). A lung/liver-on-a-chip platform for acute and chronic toxicity studies. Lab on a Chip, 18(24), 3814–3829. https://doi.org/10.1039/c8lc01029c
- Low, L. A., Mummery, C., Berridge, B. R., Austin, C. P., & Tagle, D. A. (2020). Organs-on- chips: into the next decade. Nature Reviews Drug Discovery. doi:10.1038/s41573-020-0079-3