Imagine yourself amidst a bustling crowd of people. You’re interacting, socializing, and having conversations with people, when suddenly someone sneezes–ACHOO! They say “excuse me,” and you reply with “bless you;” a routine exchange of courtesy has occurred, and you don’t give their sneeze a second thought. Unfortunately, they were sick, and their sneeze droplets entered your nostril. My goal in setting this scene is not to make you hyper-aware and paranoid about people sneezing–I think we’ve all heard enough about transmissibility through coughs and sneezes over the course of this pandemic. However, I do want to talk about how your body defends itself against the millions of foreign microorganisms that enter it every day.
We have three main lines of defense against any pathogen that attempts to infect our body. Picture your body as a kingdom–the first line of defense is the physical barrier created by our skin and the mucus lining of the digestive and respiratory tracts. You can think of this as the moat that prevents invaders from entering the kingdom. The skin acts as an impermeable barrier to pathogens, while the stickiness of the mucosa traps pathogens. Other examples of physical barriers include the hair that lines our nasal and ear canals, as well as ear wax. The low pH atmosphere created by gastric juice and urine also lyse many pathogens. When these physical barriers fail, our body has a non-specific innate response that involves immune cells–you can think of this immune response as the guards who stand at the gates of the castle, marching around the kingdom looking for invaders. Known as phagocytes, these immune cells recognize and eliminate pathogens. This process is called phagocytosis, in which the phagocytes bind to the pathogen, engulf it, and digest it. Finally the third line of defense, called the specific adaptive response, is taken on by the most skilled guards. This defense involves two types of lymphocytes (white blood cells): B lymphocytes and T lymphocytes. When our body encounters a pathogen that we have encountered before, our immune system uses its memory cells which produce antibodies to specifically target that pathogen.
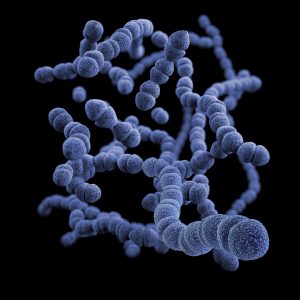
But where do these immune cells come from? All cellular components of our blood, including phagocytes called macrophages, originate from specialized hematopoietic stem cells contained in the body’s bone marrow. Macrophages are specialized fighter cells that perform the detection, phagocytosis, and destruction of pathogens. These macrophages are distributed widely throughout our bodily tissues and constantly circulate in our blood vessels. They march around the kingdom, guarding it against foreign invaders. Macrophages continuously multiply as they travel through the body and are primarily responsible for our innate nonspecific immune response.
To effectively combat infections and tumors, macrophages need to infiltrate cell tissue and wait for an opportune time to attack. Despite their importance, the mechanisms that immune cells use to squeeze through tissue cell barriers were a scientific mystery for many years. How do the macrophages not get crushed while squeezing through cell tissue?
In order to study this cell invasion process in detail, Professor Daria Siekhaus and her team at the Institute of Science and Technology Austria turned to Drosophila (fruit fly) embryos to use as a model. During development, Drosophila embryos are transparent and predominantly protected by macrophages, making them the perfect specimens to study macrophage activity. Using bioimaging technology, the researchers marked macrophages with a green fluorescent protein and tracked their movement from where they were created to where they fight off pathogens.
They found a gene called Dfos that triggers the assembly of actin filaments inside the cell membrane of the macrophage. These actin filaments thicken due to a complex cascade of many protein interactions and act as an armor to protect the macrophage from damage. The actin filaments form a stable shell around the macrophage and deform any cells in their path–like a knight’s shield and armor. The scientists found that the actin shell was integral to macrophage function when they ran the same experiment in vivo, but altered the protein interactions to prevent the formation of the denser, connected actin filaments. Here, they found that the macrophages could not advance through cell tissue unless their surroundings were softened. Once the macrophages complete their quest to find and kill pathogens, the actin filaments disassemble and become loose in structure to regain their malleability. Now you might be wondering, how does a study done on fruit fly embryos translate into learning more about the human immune system? It turns out that the Dfos gene in fruit flies is very similar to the Fos gene in humans. They both have the same functions: to code for similar proteins that interact to assemble actin filaments!
Once the macrophages have reached their target pathogen, they begin to engulf the pathogen, curving their malleable cell walls around the invader. Once engulfed, a separate internal compartment called a phagosome is created, which proceeds to fuse with the lysosome (a cellular compartment in the macrophage that breaks down invading viruses and bacteria). The digestive enzymes in the lysosome destroy the pathogen by breaking down its cell membrane and structure into tiny pieces. These toxic pieces are then expelled from the macrophage and destroyed by T-cells (another type of immune cell that partakes in specific adaptive immune response).
But how do we benefit from knowing the complex mechanisms of how immune cells travel in the body? It turns out that like immune cells, cancer cells also need to squeeze between connected cell tissue to disseminate throughout the body. Thus, Siekhaus used her research about macrophages to propel new investigations in oncology. Many types of immune cells infiltrate tumors and can either promote or impede the advancing and metastasizing of cancer cells. Using a combination of imaging, genetics, cell biology, biophysics, and modeling, researchers have found a cytokine in vertebrates — small proteins important in cell signaling — that facilitates tissue entry by softening surrounding tissues. They have also identified an invader program in macrophages that activates the transcription and translation of an mRNA subset to increase tissue entry, thereby facilitating cancer cells to further metastasize. The team is currently researching if certain shifts in cell surface actin properties can be useful in impeding the dissemination of cancer cells.
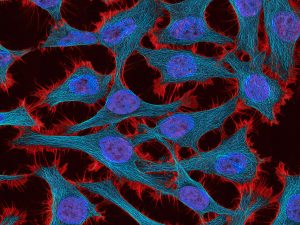
Our bodies are at a never-ending war with their enemies, the pathogen, determined to win every battle. But we only have a finite number of guards at our disposal, and oftentimes we are overpowered by our enemies. Hence, understanding how our immune system behaves, what pathways it utilizes, and how it combats pathogens is vital information to propel novel research in identifying targeted treatments for cancer.
References:
- https://www.news-medical.net/health/What-are-the-Three-Lines-of-Defense.aspx#:~:text=The%20immune%20system’s%20three%20lines,responses%2C%20and%20specific%20adaptive%20responses.
- https://www.technologynetworks.com/cell-science/news/mechanism-that-helps-immune-cells-to-invade-tissues-discovered-357332
- https://www.sciencetimes.com/articles/35431/20220107/cell-invasion-scientists-discover-mechanisms-help-immune-cells-invade-tissues.htm
- https://journals.plos.org/plosbiology/article?id=10.1371/journal.pbio.3001494
- https://www.ncbi.nlm.nih.gov/books/NBK27092/#:~:text=The%20cells%20of%20the%20immune,vessels%20called%20the%20lymphatic%20system.
- https://ist.ac.at/en/research/siekhaus-group/
- https://unsplash.com/