ABSTRACT
Urokinase-type plasminogen activator (uPA) is a serine protease responsible for cleaving and activating inactive plasminogen to its active form, plasmin, which is involved in fibrinolysis, angiogenesis, and extracellular matrix degradation. Plasmin, in turn, is a uPA zymogen activator. Hence, the plasmin-uPA system is a positive feedback loop. The catalytic activity of uPA is influenced by the orientation of its catalytic triad and the substrate-binding affinity at the uPA’s specificity pocket. The enzyme consists of an amino-terminal fragment (ATF) connected to the uPA protease domain via a disulfide bond and a disordered linker. We wanted to examine the effect the ATF has on the catalytic activity of the uPA protease. We hypothesized that the presence of the ATF enhances the activity, increases the inhibitory capacity (how much inhibitor uPA can tolerate), and alters the dynamics of the uPA protease. In this study, the activity and inhibition of full-length human uPA (huPA) with the ATF, huPA with an N-terminal polyhistidine tag replacing the ATF (huPA protease with N-terminal His-tag), and huPA without ATF entirely (huPA protease), were compared with and without the inhibitor benzamidine. Our results showed that the catalytic efficiency (kcat/Km) of full-length huPA and huPA protease with N-terminal His-tag are fourfold and threefold higher than that of huPA protease, respectively. Furthermore, the uPA inhibitor, benzamidine’s IC50 (inhibitor concentration needed to inhibit uPA to half its uninhibited activity) for the full-length huPA and the huPA protease with N-terminal His-tag are fivefold and twofold higher than that of the protease domain only, respectively. These results suggest that the presence of the ATF enhances the catalytic activity of huPA and reduces benzamidine’s capacity to inhibit huPA. Moreover, the N-terminal His-tag, like the ATF, seems to partially enhance huPA activity. By scrutinizing the significance of the ATF to the activity of huPA, we can introduce more research questions about how the uPA-plasmin system can be regulated in our physiological systems.
INTRODUCTION
Urokinase-type plasminogen activator (uPA) is a serine protease responsible for cleaving and activating the inactive plasminogen to active plasmin. The plasmin-uPA system is largely involved in regulating the degradation of the extracellular matrix and fibrin blood clots as well as angiogenesis, which is the formation of new blood vessels.¹,² Deregulation of the uPA-plasmin system can contribute to tumor progression caused by factors such as tumor angiogenesis.³,⁴
uPA consists of an N-terminal Epidermal Growth Factor-like (EGF-like) domain, a Kringle domain, a disordered linker region, and a protease domain. The EGF-like domain and the Kringle domain collectively comprise the amino-terminal fragment (ATF).⁵ The linker region connects the ATF to the C-terminal catalytic protease domain of the uPA (Fig 1). In its zymogen form, uPA is one single chain. Active uPA after plasmin cleavage consists of two chains: the ATF-linker and the catalytic protease, which are held together via a disulfide bond.² Previous hydrogen-deuterium exchange mass spectrometry (HDX-MS) data from our lab revealed that although the ATF is distant from the protease domain, its presence makes regions of the protease more rigid (less dynamic). Higher rigidity in certain uPA regions indicate that the regions are more “stuck” in a certain conformation. Thus, the presence of the ATF may play a role in the dynamic allostery, or the modulation of conformational dynamics of the uPA protease from a distance. Additionally, our lab also showed that when the ATF is present, uPA shows an increase in catalysis (Fig 2). In the crystal structure of the protease domain of uPA (PDB: 1EJN), we can see the catalytic triad (His57, Asp102 and Ser195) which is responsible for cleaving substrates and the approximate specificity pocket region (around Asp189) which is where substrates would bind. The activity of uPA results from both the catalytic efficiency of the catalytic triad and the substrate-binding affinity at the specificity pocket. Thus, the presence of the ATF seems to affect both of the mentioned regions of the protease domain.
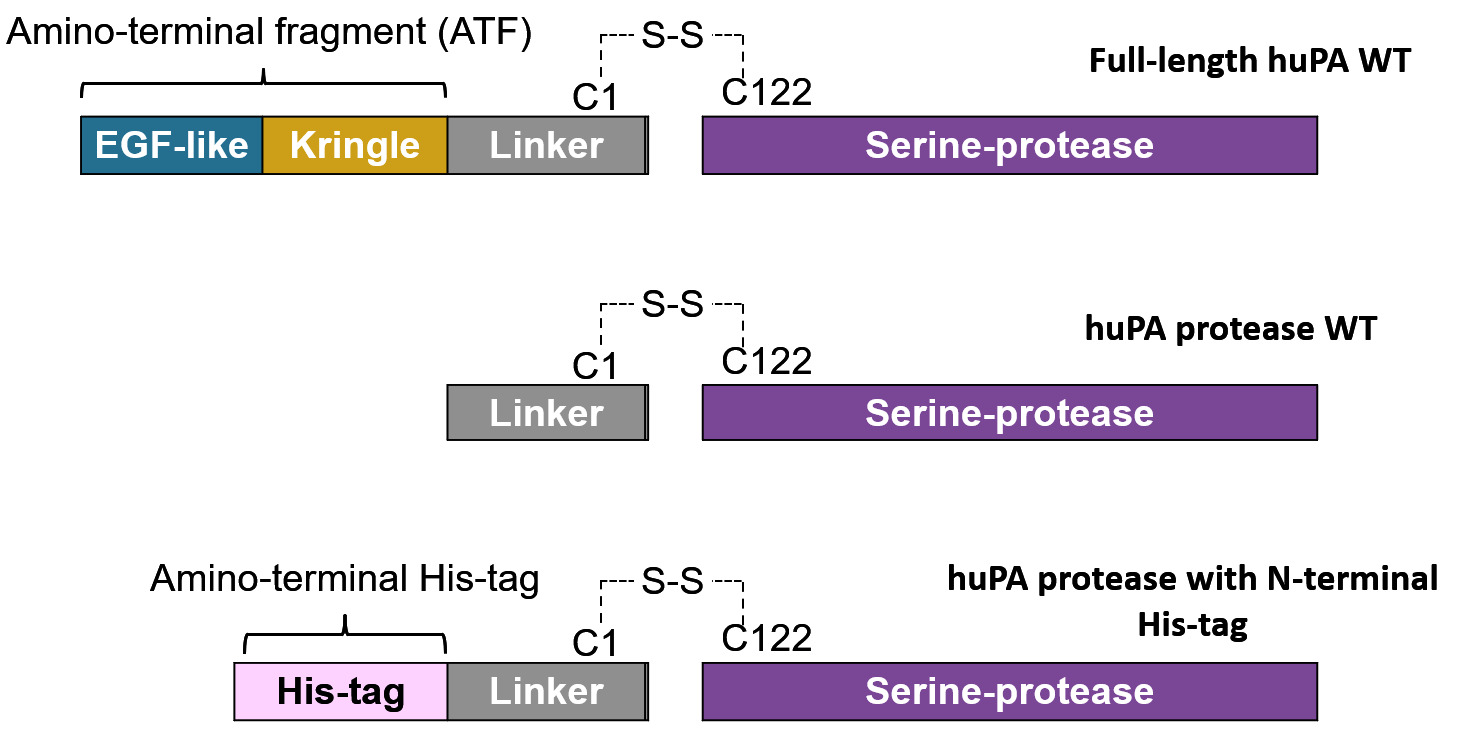
As most of the current research on uPA scrutinizes the uPA protease domain only, we wanted to investigate whether the presence of the ATF alters the activity and inhibitory capacity of huPA. Understanding how the ATF influences the activity and inhibition of huPA would allow us to better understand and pose more questions regarding the role of the ATF in huPA. While huPA activity results from both the activity of the catalytic triad and substrate binding specificity at the specificity pocket, inhibition assays will depict the ATF’s particular effects on the inhibitor and substrate binding specificity pocket.⁶ We examined full-length huPA which consists of the entire ATF-linker-protease and compared it to huPA protease which consists of only the linker-protease. In addition, we also examined huPA protease with an N-terminal His-tag in the place of the ATF to see whether the activity and inhibition of the enzyme are altered.
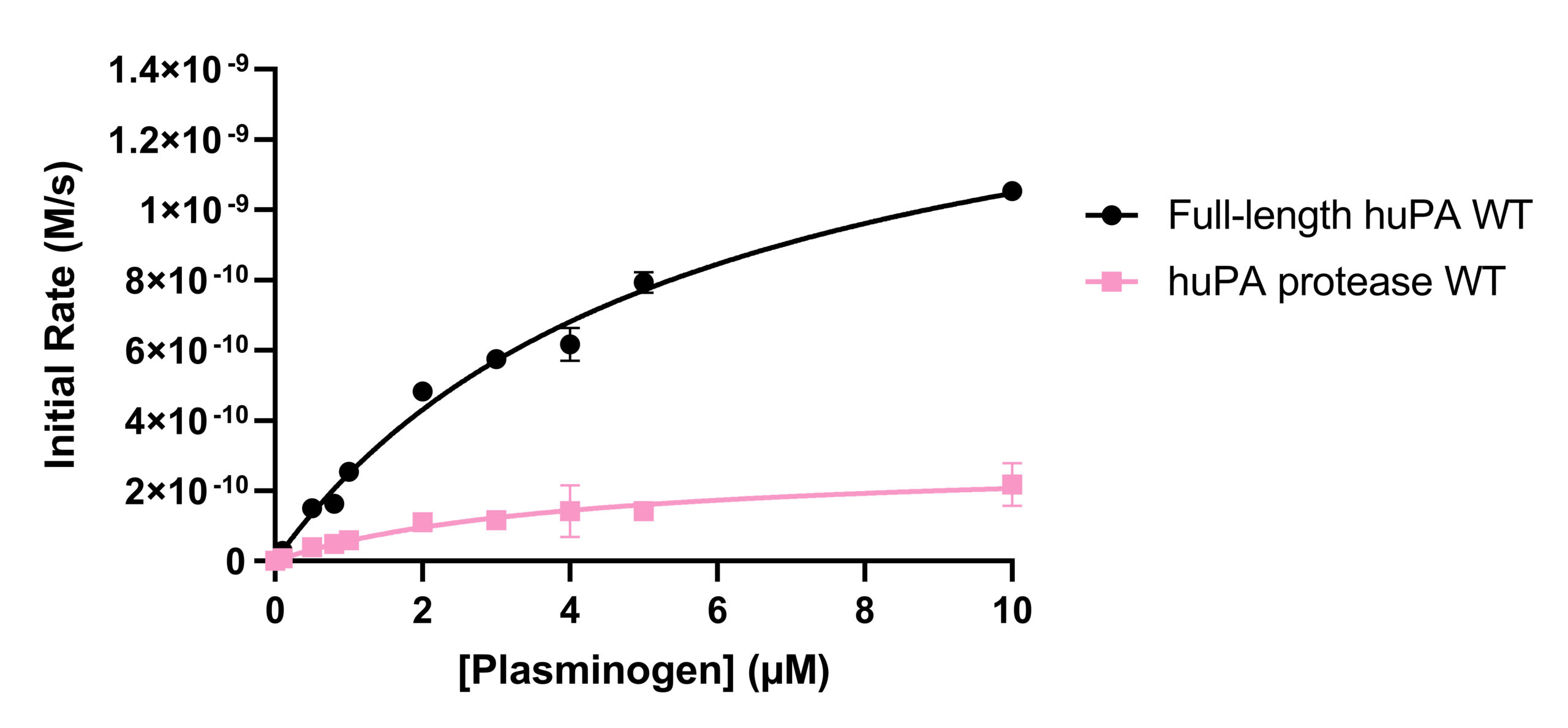
We hypothesize that the ATF can stabilize the uPA protease by increasing its rigidity and determining its dynamics. Particularly, with the ATF, the uPA catalytic triad would more likely be in the active state conformation, thus it would be more frequent and effective at cleaving its substrate. In the case of the huPA protease, we hypothesize that because there is no ATF present, the protease domain responsible for catalysis is more dynamic (frequent movements), so the catalytic triad is less frequently in the correct conformation for substrate cleavage. Furthermore, the ATF may play a role in increasing the substrate specificity and affinity of uPA’s substrate-binding pocket in the uPA protease domain, making full-length huPA more selective.
MATERIAL AND METHODS
Purification of Full-Length huPA, huPA protease, and huPA protease with N-terminal His-tag
To test whether the addition of the ATF impacted catalytic ability, we made the enzyme using the following approach. Fulllength huPA, huPA protease, and huPA protease with N-terminal His-tag were expressed as inclusion bodies in BL21 (DE3) competent E. coli cells. To properly refold the proteins and form the correct disulfide bonds, the E. coli cell pellets were resuspended in sonication buffer (0.5 M NaCl; 50 mM Tris-HCl pH 8; 1 mM EDTA pH 8; 10% glycerol by volume; 1 mM β-mercaptoethanol; 1% Triton X by volume) and sonicated on ice (amplitude 50). 300 μg DNAse I, 300 μL 1 M MgCl2, and 300 μL 1 M MnCl2 were added to the lysed cells, which were then incubated while rocking at room temperature. The sonicated cell lysate was treated with DNAse for 45 minutes and then centrifuged at 17,000 RCF, 4 °C for 15 minutes. The pellets were washed once with a modified sonication buffer with 0.25% Triton X by volume, and once with another modified sonication buffer without Triton X. With each wash, the pellet was resuspended and centrifuged at 17,000 RCF, 4°C for 10 minutes. The washed inclusion bodies were then resuspended in denaturing buffer 1 (6 M Urea, 100 mM NaCl; 50 mM Tris-HCl pH 8; 1 mMradation during storage). When ready for activity and inhibition assays, fresh huPA proteins were size excluded with a Superdex 75 column equilibrated with 1X PBS at pH 7.4. Fractions collected for the corresponding huPA protein peak were pooled together, concentrated, and used for activity and inhibition assays.
Activity Assays with Chromogenic Substrates
To evaluate the activity of full-length huPA, huPA protease, and huPA protease with N-terminal His-tag, 10 nM enzyme was incubated for 10 minutes in 1X PBS pH 7.4 with various concentrations of substrates at 37 °C using 96-well microtiter plates. After adding huPA, the initial velocities of the chromogenic substrate cleavage resulting in p-nitroaniline (pNA) formation were monitored for 10 minutes at 37 °C at an absorbance of 405 nm in a microplate reader. The two huPA substrates used were S-2444 and Glu-plasminogen.
S-2444 (Pyro-Glu-Gly-Arg-pNA) (DiaPharma): The concentrations of S-2444 used were 0 μM to 500 μM. When S-2444 is hydrolyzed by huPA, pNA is produced. The product absorbs light at 405 nm, thus pNA formation can be monitored with absorbance at 405 nm to quantify the initial velocity of the huPA enzymes.
The absorbance data was converted to the velocity of product formation (initial velocity of enzyme); the maximum reaction rate (Vmax) and Michaelis constant (Km) values were determined by fitting the data to the Michaelis-Menten kinetics equation in GraphPad Prism. The turnover number (kcat) and the catalytic efficiency of enzymes (kcat/Km) were determined with the corresponding Vmax and Km values.
Glu-plasminogen (Haematologic Technologies): The concentrations of Glu-plasminogen used ranged from 0 μM to 10 μM. The chromogenic substrate for active plasmin is S-2251 (DiaPharma). When Glu-plasminogen is activated to plasmin by huPA, plasmin hydrolyzes S-2251 and produces pNA. pNA formation was monitored with absorbance at 405 nm to quantify the initial velocity of plasmin and therefore the initial velocity of the huPA enzymes.
Inhibition Assays with Benzamidine and UK-122
Benzamidine: To evaluate how full-length huPA, huPA protease, and huPA protease with N-terminal His-tag are inhibited by competitive inhibitors, 10 nM huPA enzymes were incubated with various concentrations of the inhibitor at 37 °C in 1X PBS for 10 minutes on 96-well microtiter plates. Then, S-2444 was added to a final concentration of 75 μM in the reaction. The initial velocities of the chromogenic substrates cleavage to pNA by huPA enzymes were monitored for 10 minutes at 37 °C with an absorbance of 405 nm in the microplate reader. The IC50 values for the huPA constructs were calculated using GraphPad Prism by fitting the normalized initial velocity data of the huPA enzymes to the equation. Dose-response – Inhibition: [inhibitor] vs. normalized response – variable slope. The normalized data (percent activity of the huPA proteins) were calculated based on the initial velocity of the huPA proteins when no inhibitor was added (100 percent activity).
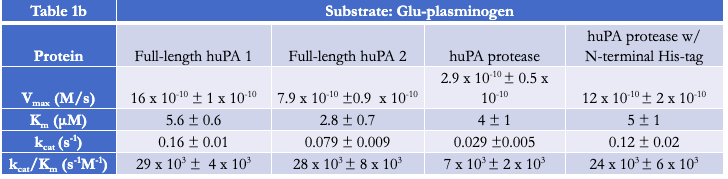
UK-122 inhibition: UK-122 was prepared in dimethyl sulfoxide (DMSO). 10 nM huPA enzymes were incubated with different concentrations of UK-122 at 37 °C in 1X PBS, 5% DMSO, and 10% BSA for 10 minutes. As UK-122 is colored, a control well was implemented using all the reagents that comprise the experimental wells, except the huPA enzymes. The absorbances from the control wells were subtracted from every corresponding experimental well.
DATA AND RESULTS
Activity and inhibition assay analyses were performed with both full-length huPA (with ATF) and huPA protease (without ATF). Our lab has previously shown that with the S2444 and Glu-plasminogen huPA substrates, there appear to be differences between the activity of full-length huPA and huPA protease. The difference is more apparent in the Glu-plasminogen activity assay, where the Vmax of the huPA protease showed an approximate sixfold decrease in comparison to that of the full-length huPA (Fig 2b). Additionally, the kcat/Km value of full-length huPA is approximately fourfold higher than that of huPA protease (Table 1b). On the other hand, the rate of the hydrolysis of full-length huPA is approximately one and a half times lower than that of huPA protease in the S-2444 activity assay (Table 1a). The activity assays showcase enzyme differences in both the catalytic triad dynamics (how often the triad is in the correct catalytic orientation to hydrolyze the substrate) and the substrate binding specificity at the specificity pocket. Thus, it is worth investigating how the two protein constructs differ specifically at the specificity pocket with inhibition assays. As inhibition assays focus on binding of inhibition substrates at the specificity pocket, we used this method to compare full-length huPA and huPA protease to elucidate the effect of ATF on the huPA protease domain.
Activity Assays with Chromogenic Substrate S-2444 and Substrate Glu-plasminogen
Activity assays of full-length huPA and huPA protease with N-terminal His-tag were performed with the chromogenic substrate S-2444 and substrate Glu-plasminogen (Fig 3a-3b). The activity of the full-length huPA and huPA protease with N-terminal His-tag are approximately identical. The kcat/Km values of full-length huPA and huPA protease with N-terminal His-tag are very similar in both the S-2444 and Glu-plasminogen activity assays. The kcat/Km value of huPA protease is more than threefold lower than that of huPA protease with N-terminal His-tag (Table 1a-1b). When superimposed, we can clearly see the activity of huPA protease with N-terminal His-tag is only slightly lower than the activity of full-length huPA (Fig 3c).
Inhibition Assays with uPA Inhibitor Benzamidine
Inhibition assays of full-length huPA, huPA protease, and huPA protease with N-terminal His-tag were performed with S-2444 as the chromogenic substrate to measure huPA activity, and benzamidine as the competitive inhibitor to monitor inhibition (Fig 4). The corresponding IC50 values, the concentration of benzamidine required for the enzyme to be inhibited by 50% at 75 μM S-2444, are indicated in Table 2. Full-length huPA has the highest IC50, approximately twofold higher than the IC50 of huPA protease with N-terminal His-tag and approximately five-fold higher than that of huPA protease. Based on the inhibition assay curves, we can see that the inhibition of full-length huPA has a higher percent activity (~25%) than huPA proteases (~15%). Although the IC50 of huPA protease is higher than that of huPA protease with N-terminal His-tag, the inhibition curves plateau at similar percent activity values.

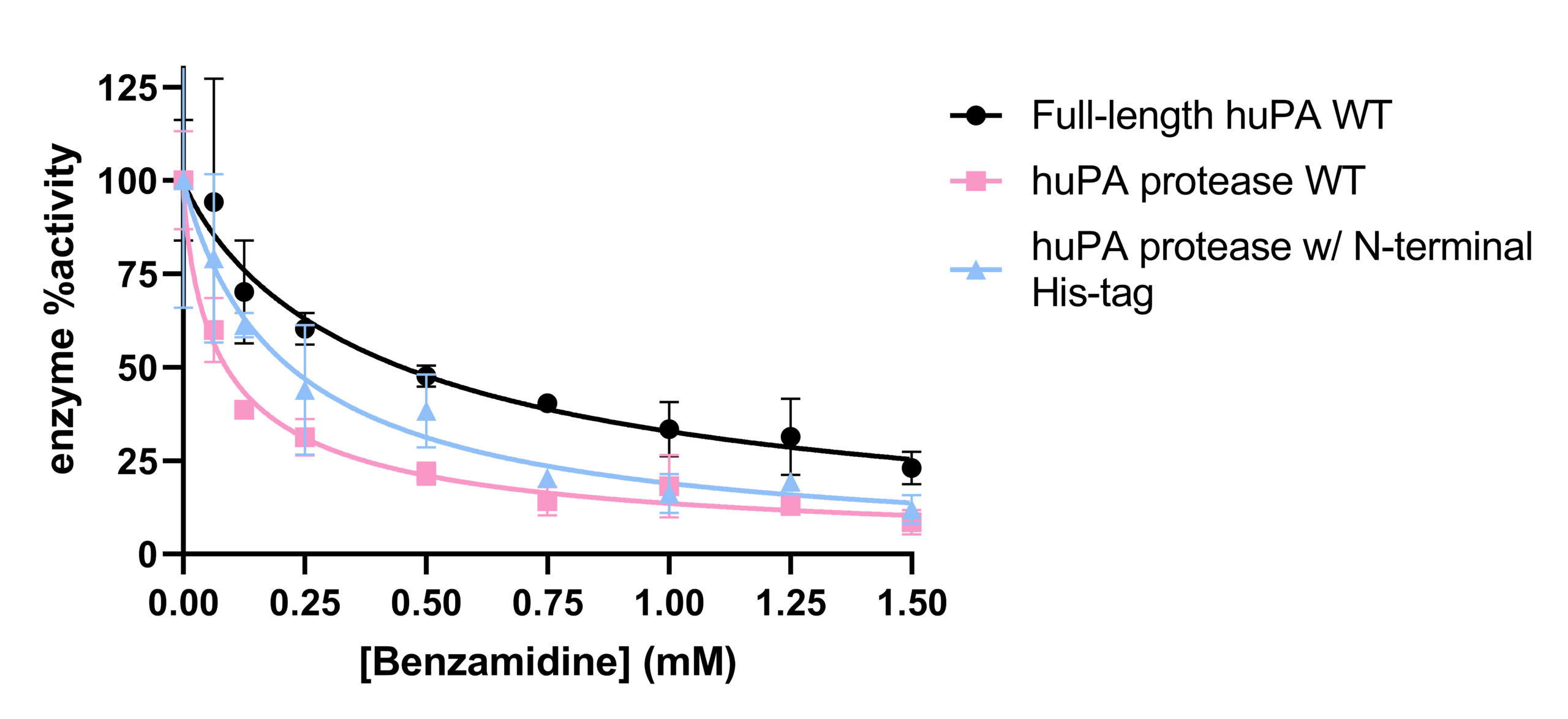

Inhibition Assays with uPA Inhibitor UK-122
UK-122 is a larger and more specific competitive inhibitor than benzamidine.⁷ Inhibition assays of full-length huPA and huPA protease with N-terminal His-tag using UK-122 as the competitive inhibitor and S-2444 as the chromogenic substrate were performed and are in progress of standardization. We started the UK-122 inhibition assay with full-length huPA with a low range of UK-122 concentration (0 to 500 nM) because the IC50 of uPA for UK-122 is 200 nM.7 However, the range of UK-122 was too low and narrow, as full-length huPA activity only went down to approximately 75% (Fig 5a). Next, we broadened the UK-122 concentration range and performed the inhibition assay with huPA protease with N-terminal His-tag (Fig 5b) and obtained its IC50 for UK-122 (Table 2).
DISCUSSION
Previous experiments with Glu-plasminogen activity assays demonstrated that full-length huPA has higher activity than huPA protease (unpublished data by Constanza Torres Paris). These findings evoked the research question regarding the effects ATF may have on the activity (catalytic triad dynamics and substrate affinity at the specificity pocket) and inhibition (inhibition and substrate binding affinity at the specificity pocket) of huPA. On the other hand, the differences between full-length huPA and huPA protease were not as apparent in S-2444 activity assays. The differences in the results from the Glu-plasminogen and S-2444 activity assays provoke questions as to why different substrates show different uPA activity changes in the uPA constructs used in this study. Plasminogen (molecular weight: 88,000 g/mol) is a much bigger substrate for uPA than S-2444 (molecular weight: 489.9 g/mol). We are interested in investigating whether the presence of the ATF impacts the catalytic triad orientation and the specificity pocket, as well as the ability to identify these effects.
The Glu-plasminogen and S-2444 activity assays both showed no significant difference between the hydrolysis rates of the full-length huPA and huPA protease with N-terminal His-tag (Fig 3). For the Glu-plasminogen assay in particular, the activity of huPA protease with N-terminal His-tag is more than threefold greater than the activity of huPA protease, which has no His-tag in the place of the ATF. The data suggests that having the N-terminal His-tag in the place of the ATF influenced the activity and inhibition of huPA. In other words, when the His-tag is attached to the N-terminal side of the huPA protease in place of the ATF, huPA activity is improved. This is shown by an increase in Vmax and kcat/Km in comparison to huPA protease. It is possible that the ATF enhances the efficiency of the uPA protease domain through an allosteric effect on the protease domain via the linker. However, the effect of the N-terminal His-tag on huPA protease is not apparent in the results obtained from S-2444 activity assays. This may be due to the differences in the substrates S-2444 and Glu-plasminogen, as plasminogen is a much bigger huPA substrate than S-2444. The presence of the N-terminal ATF and
N-terminal His-tag may have contributed to a higher substrate binding affinity for plasminogen, the natural substrate of huPA. The enhancement of specificity may not have been as apparent for the synthetic chromogenic substrate S-2444. However, since activity assays look at both the catalytic triad efficiency and the specificity pocket, the differences between full-length huPA and huPA protease may have resulted from a difference in the catalytic efficiency of the catalytic triad as well. Thus, inhibition assays may be able to tell us more about what is happening at the specificity pocket of the uPA protease.
Inhibition assays using benzamidine as the inhibitor and S-2444 as the huPA substrate were performed. It was hypothesized that full-length huPA will bind weaker to benzamidine because it is likely more specific to its substrates than a general serine protease inhibitor. According to the IC50 values in Table 2, full-length huPA requires a fivefold higher concentration of benzamidine than huPA protease to have its activity inhibited by 50%. huPA protease with N-terminal His-tag also requires more than twofold more benzamidine than huPA protease to inhibit its activity by 50%. These findings suggest that full-length huPA binds weaker to the competitive inhibitor benzamidine than the other two uPA constructs. Interestingly, huPA protease with N-termial His-tag also requires more than twofold more benzamidine than huPA protease to inhibit its activity by 50%. These findings suggest that full-length huPA binds weaker to the competitive inhibitor benzamidine than the other two uPA constructs. Interestingly, huPA protease with N-terminal His-tag experienced less inhibition than huPA protease. Collectively, these inhibition assays suggest that the presence of the ATF enhances the substrate binding affinity for S-2444 more than the presence of the His-tag at the specificity pocket of the huPA protease domain. Benzamidine-Glu-plasminogen inhibition assays were unfeasible because benzamidine will inhibit the plasmin when plasminogen is activated.1 Thus, we will not be able to measure huPA’s activity if plasmin cannot hydrolyze its chromogenic substrate, S-2251, to show uPA activity (Fig 2b). Benzamidine is a very small inhibitor in comparison to plasminogen, the natural substrate for huPA. Moreover, benzamidine is also a very broad inhibitor with low specificity to huPA. The findings from benzamidine inhibition assays provoke the question of whether other inhibitors with variations in sizes and specificity will show similar results when investigating the impact of ATF on uPA.
UK-122 is a synthetic inhibitor which was reported to have a high specificity for uPA, with an IC50 of 0.2 μM.2 The IC50 obtained from our experiments on huPA protease, however, was 96 μM (reported as 0.10 ± 0.01 mM in Table 2). Our results show that UK-122 binds weaker to both full-length huPA and huPA protease compared to what was previously reported. In addition, the binding between UK-122 and full-length huPA was too weak to calculate an IC50. We were surprised to find a discrepancy between our results and the previously reported IC50 of 0.2 μM.7 It is worth noting that when UK-122 was first synthesized and tested, purified full-length huPA was reportedly used by American Diagnostica.⁷ Based on our experiments we learned that active full-length huPA quickly undergoes autocatalytic cleavage into huPA protease by cleaving off the ATF. Thus, the experimenters who developed and tested UK-122 could have been testing the inhibitor with huPA protease instead of full-length huPA; we plan to measure the UK-122 inhibition assays in the future to evaluate these findings. Moreover, we will test UK-122 for inhibition with Glu-plasminogen as the substrate because UK-122 is reported to be specific for uPA and does not inhibit plasmin at the UK-122 concentrations we will be using in our assays.⁷ It is possible that UK-122 is a better inhibitor against plasminogen than against S-2444. As all of the assays done in this project were performed with freshly purified huPA proteins, the findings discovered in this project push for future investigations into the differences between full-length huPA and huPA protease.
CONCLUSION
Our results showed that the kcat/Km, of the full-length huPA is fourfold higher than that of the huPA protease alone, while the huPA protease with N-terminal His-tag had a kcat/Km threefold higher than that of huPA protease. On the other hand, the IC50 of benzamidine on full-length huPA is fivefold higher than that of the huPA protease alone, while the IC50 of huPA protease with an N-terminal His-tag is twofold higher than that of the huPA protease alone. These results indicate that the presence of the ATF does affect huPA activity. In particular, the ATF enhances catalytic activity and reduces the capacity of benzamidine to inhibit uPA. Interestingly, the N-terminal His-tag appears to serve a role in partially stabilizing the protease in the place of the ATF. Studying the ATF’s effect on uPA activity gives insight as to how uPA activates plasmin. This observation facilitates an improved comprehension of plasmin’s involvement in the corresponding physiological processes such as regulating the degradation of the extracellular matrix and fibrin blood clots. Additionally, uPA activity is closely related to unregulated involvement of plasmin in angiogenesis, a significant pathological phenomenon under study.
In the future, we plan to reproduce and optimize the inhibition and activity assays with UK-122 and new uPA inhibitors and activators, including ATP (inhibitor) and polyphosphates (activator), that are present in natural blood clot environments. By looking at more inhibitors with different sizes and uPA specificities, we can take a closer look at how the ATF influences the specificity pocket of huPA. After comparing the inhibition assay data from full-length huPA and huPA protease, we also plan to perform HDX-MS to scrutinize the change in dynamics between the various huPA constructs and map where these compounds are binding to the uPA.
ACKNOWLEDGEMENTS
I acknowledge the UC San Diego Komives Lab. Specifically, this work was supervised and supported by my Principal Investigator Dr. Elizabeth Komives and graduate student mentor Constanza Torres Paris. This work was funded by the Undergraduate Research Scholarships (URS) – Eureka! Scholarship for Biological Sciences Majors, which was sponsored by Dr. Wendy Kwok. Additionally, I would like to acknowledge the Triton Research & Experiential Learning Scholars (TRELS) Quarterly Program as well as the Undergraduate Research Hub at UCSD.
REFERENCES
- Andreasen, P. A., Kjoller, L., Christensen, L. & Duffy, M. J. The urokinase-type plasminogen activator system in cancer metastasis: a review. Int J Cancer 72, 1–22 (1997).
- Andreasen, P. A., Egelund, R. & Petersen, H. H. The plasminogen activation system in tumor growth, invasion, and metastasis. Cell Mol Life Sci 57, 25–40 (2000).
- Dano, K. et al. Plasminogen activators, tissue degradation, and cancer. Adv Cancer Res 44, 139–266 (1985).
- Deryugina, E. I., & Quigley, J. P.. Cell surface remodeling by plasmin: a new function for an old enzyme. Journal of biomedicine & biotechnology, 2012, 564259.
- Barinka, C. et al.. Structural basis of interaction between urokinase-type plasminogen activator and its receptor. Journal of molecular biology, 363(2), 482–495 (2006).
- Hedstrom, L.. Serine protease mechanism and specificity. Chemical Reviews, 102(12), 4501–4523 (2002).
- Zhu, M. et al.. Identification of a novel inhibitor of urokinase-type plasminogen activator. Molecular Cancer Therapeutics, 6(4), 1348–1356 (2007).
- Kromann-Hansen, T. et al.. Ligand binding modulates the structural dynamics and activity of urokinase-Type plasminogen activator: A possible mechanism of plasminogen activation. PLoS ONE, 13(2), 1–16 (2018).
- Kromann-Hansen, T., Lund, I. K., Liu, Z., Andreasen, P. A., Høyer-Hansen, G., & Sørensen, H. P.. Allosteric inactivation of a trypsin-like serine protease by an antibody binding to the 37- and 70-Loops. Biochemistry, 52(40) (2013)
- Alves, N. J., & Kline, J. A.. Comparative study on the inhibition of plasmin and delta-plasmin via benzamidine derivatives. Biochemical and Biophysical Research Communications, 457(3), 358–362 (2015).
- Papaleo, E. et al.. The role of protein loops and linkers in conformational dynamics and allostery. Chemical Reviews, 116(11), 6391–6423 (2016).
- Sperl, S. et al.. (4-Aminomethyl)phenylguanidine derivatives as nonpeptidic highly selective inhibitors of human urokinase. Proceedings of the National Academy of Sciences of the United States of America, 97(10), 5113–5118 (2000).
Written by Harriet J. Song [1], Constanza Torres-Paris [2], and Elizabeth A. Komives
[1] Division of Biological Sciences, University of California San Diego, Earl Warren College, Molecular and Cell Biology Major, Class of 2022, La Jolla, California, United States, [2] Department of Chemistry and Biochemistry, University of California, San Diego, La Jolla, California, United States