Imagine: it’s Friday morning, and you have awoken from your slumber to one of the cloudier days in La Jolla. A perfect day for you to finally open up the box of mint tea bags that you bought on wholesale last weekend. With the efficiency of a student who has to be in class within the next fifteen minutes, you quickly set your water to heat as you enthusiastically crack open the box of tea bags. Ding! The water heater beckons you after successfully completing its task. With a coffee mug in one hand and a tea bag in the other, you carefully, but swiftly, open the heater and pour its steaming contents into your mug. After dipping your tea bag into the hot water, you are finally ready to taste the product of your mighty efforts. Excited, you take one sip. And then you have a few more sips before you have a thought that rattles you to your core. You think to yourself: “Oh my! My hot tea tastes… cold?”
How is it possible to taste coolness? How is it possible to taste temperature at all? In order to answer this question, we must first understand how sensory input is processed. There are two different pathways through which processing occurs: one that encodes information relating to pain or temperature (noxious) and one that encodes all other sensory information (non-noxious). Once a sensory stimulus is recognized by the sensory receptors of the body, the sensory information takes either the noxious or the non-noxious pathway to the brain (where the information is processed). The pathway taken is dependent on the specific type of sensory receptors that are activated by the stimulus itself.
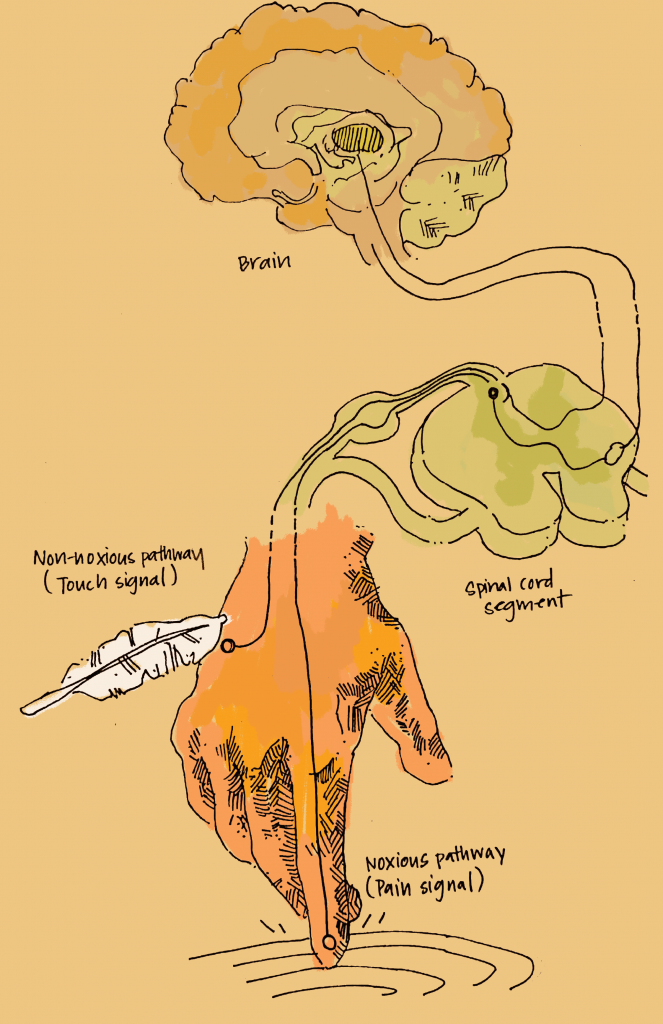
For example, touching a scorching hot pan on a stove will activate one type of receptor on a sensory neuron, such as a vanilloid receptor. Activating this receptor may cause an influx or an efflux of ions that will change the membrane potential (the difference between the potential inside and outside the neuron) of the neuron, as the incoming or outgoing ions will have either positive or negative charges. This will then cause electrical signals (in the form of action potentials) to fire between neurons throughout the noxious sensory pathway: the first-order sensory neuron in the spinal cord will relay information about the stimulus to a neuron in the thalamus of the brain, which will then relay the information to a neuron in the cortex. Once the sensory information has been relayed to the cortex, pain is perceived.
On the contrary, a non-painful stimulus (for example, touching the surface of a desk) will activate the receptive field of a different type of sensory neuron, such as a Merkel’s disc. This receptor, when activated by the stimulus of touch, will allow the neuron to fire signals that travel through the other pathway of the sensory nervous system–the non-noxious pathway. The neuron in the cortex that is activated through this relay of information will then process the stimulus as a non-painful, non-temperature-sensitive one. It is clear that the pathway taken by the electrical signals stimulates a specific neuron in the cortex, ultimately determining whether a stimulus is perceived as painful or not.
Furthermore, if a harmless stimulus activates a receptor of the noxious pathway rather than the non-noxious pathway, the cortex can be tricked into believing that a stimulus is noxious. This is the mechanism used by chemicals in certain foods that allow you to taste temperature; these substances bind to receptors of the noxious pathway, thus leading to perceived pain or temperature.
One of the most well-known compounds that behaves in this way is capsaicin, which is found in many peppers. The familiar sensation of one’s mouth catching fire after a bite of a chili pepper is a result of this compound. This burning feeling occurs because of a biochemical anomaly: capsaicin binding on to a Vanilloid receptor, one of the receptors in the pain/temperature pathway. Usually, Vanilloid receptors detect heat and send signals to the cortex through the noxious pathway to indicate its presence. However, the biochemistry of capsaicin allows it to bind to Vanilloid receptors, which results in a conformational change in these receptors. This change in shape allows for the influx of sodium and calcium ions into the cell. This causes the neuron to fire action potentials that propagate and convey the electrical signals to other neurons, leading to the perception of heat in the cortex.

Just like biochemical anomalies can help explain the burning sensation produced by capsaicin, a similar phenomenon explains the perception of coolness accompanying mint. Menthol, a compound present in mint leaves, is responsible for activating TRPM8 receptors on sensory neurons of the noxious pathway. Usually, these receptors are responsible for detecting cold temperatures; however, menthol may bind to TRPM8 receptors to cause the perception of coldness. When menthol binds to this particular receptor on the tongue, the receptor allows for an influx of calcium into the cell. This influx of calcium leads to the generation of an electrical current in the cell. As electrical currents are passed from cell to cell through action potentials, the cortex perceives the following message: if the TRPM8 receptors on the surface of the tongue’s sensory neurons have been activated, then they have been exposed to cold conditions. This is how you perceive your warm mint tea to have a cold aftertaste.
So why do these mechanisms exist? From an evolutionary perspective, the biochemistry of the capsaicin and menthol compounds is advantageous for the pepper and mint plants, respectively. Since these compounds bind to receptors of sensory neurons that detect temperature and pain, the compounds can result in painful and unexpected experiences for the animals that consume the associated plants. Painful and extreme experiences with a plant would, in theory, discourage an animal from consuming the plant in the future, thus increasing the plant population’s survival rate.
The next time you take a sip of your mint tea, chew a wad of spearmint gum, or treat yourself to a scoop of mint chocolate chip ice cream, you will be able to understand why you can taste the cold temperature. Additionally, while mint tea may taste cool, you now know something much cooler: the mechanisms by which chemical compounds fool you into perceiving temperatures!
Sources:
- https://www.discovermagazine.com/health/capsaicin-02
- https://www.livescience.com/66028-why-mint-makes-your-mouth-cool.html
- https://www.scientificamerican.com/article/how-we-sense-the-heat-of-chili-peppers-and-the-cool-of-menthol-excerpt/
Illustrations by Diana Presas.